“What time is it?”It’s a question that’s heard less and less as the proliferation of smartphones puts some of the most advanced timekeeping devices in history into the hands of everyday people. The clocks on smartphones are based on signals from space, sent from the 24 GPS satellites that keep track of time by using four onboard atomic clocks. These clocks measure time based on the frequency that electrons transition between energy levels within an atom.Since they were first created in the mid-twentieth century, atomic clocks have been the gold standard of timekeeping. Indeed, the most accurate clock in the world, which is run by the National Institute of Standards and Technology in Colorado, is a ytterbium atomic clock. But now researchers around the world are working on building a better model based not on the electrons of an atom, but the nucleus.Since protons and neutrons are densely packed in the nucleus and are thus less likely to be disturbed by outside influences, researchers think the nucleus could serve as the basis for an ultra-precise atomic clock in the future. In a new paper published today in Nature, researchers in Germany describe experiments that showed for the first time what this clock would actually look like, a major milestone into making a nuclear clock a reality.In 1955, English physicists Louis Essen and Jack Parry built the first accurate atomic clock at the National Physical Laboratory in the UK. It worked by exposing cesium-133 atoms in a vacuum to microwave energy and then measuring how well the atom absorbs this microwave radiation.Electrons orbit the nucleus of an atom at certain stable energy levels that depend on the electrical properties of the nucleus itself. These orbits can be changed by adding energy to the system, which causes the electrons to temporarily get bumped up to a higher energy level and emit electromagnetic radiation during the transition. Different types of atoms are able to absorb energy at different wavelengths.
In the case of cesium-133, that wavelength is about 3.2 cm, which means the wave oscillates at a frequency of 9,192,631,770 cycles per second. When cesium-133 atoms are hit with microwaves at this frequency, it causes the atom’s single outermost electron to transition between energy states at the same rate, and it is this rapid transition that was used to formally define the length of a second in 1967. (Another way to think about this is that the cesium atom is a clock and the electron is its pendulum. In this analogy, microwave energy sets the pendulum in motion and every 9,192,631,770 swings is marked as one second on the clock face).This method of keeping time with atomic clocks has been refined over the last fifty years to the point that the most accurate clock in the world will only deviate by a second over 200 million years, but the basic principles have remained the same. Nevertheless, physicists have wondered if still more precise atomic clocks were possible by using a transition frequency in the nucleus of an atom, rather than in its electrons. The advantage of using a nucleus is that its energy transitions occur at much higher frequencies than electron transitions, which would allow for even more precise measurements of time.The difficulty with nuclear excitations, however, is that they require much higher energy levels than electron energy transitions because their protons and neutrons are more densely packed. Whereas electrons in cesium-133 can be bumped to a higher energy state at a frequency of approximately 9.1 gigahertz (on the low end of the microwave range of the electromagnetic spectrum), exciting an atom’s nucleus requires energy in the x-ray range, where frequencies range from 30 petahertz to 30 exahertz (read: 30 quadrillion to 30 quintillion cycles per second). This high energy requirement was thought to make atomic clocks based on nuclear transitions infeasible.
Yet according to a paper published today in Nature, a team of researchers at Physikalisch-Technische Bundesanstalt (PTB) in Germany may have found an exception to this rule. According to the paper, it should be possible to excite the nucleus of thorium-229 using ultraviolet light, a far more manageable energy requirement and similar lasers are already used in laser-based atomic clocks today. Moreover, the PTB team performed the first measurements that may pave the way for an atomic clock based on the energy transitions of a thorium-229 nucleus.Although ten different teams around the globe are exploring the possibility of a thorium-229 nuclear clock, progress has been slow going, because the energy level required to excite the thorium nucleus is still known only approximately. In order to make an ultra-precise nuclear clock, physicists have to dial in on the correct UV frequency. As PTB physicist Ekkehard Peik put it in a statement, finding the correct frequency “resembles the proverbial search for a needle in a haystack.”Although Peik and his colleagues haven’t yet dialed in the correct frequency to transition a thorium-229 nucleus from a ground state to an excited state, as would be the case in a future nuclear clock, their most recent paper did manage to characterize what this excited state would look like.
Advertisement
Advertisement
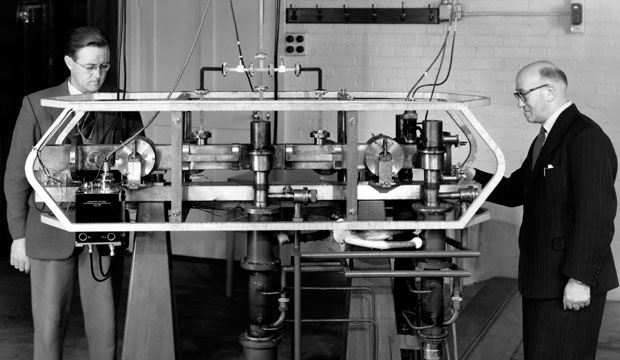
Advertisement
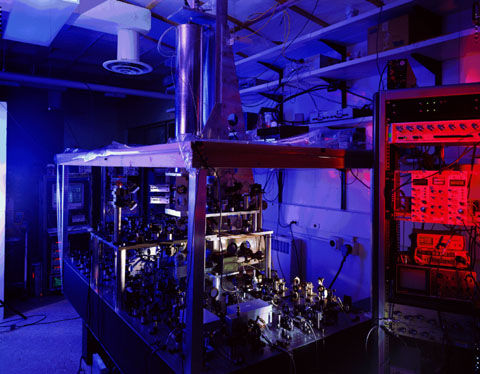
To do this, they obtained thorium-229 nuclei already in their excited state as result of alpha decay in uranium-233. These ions were then caught and stored in an ion trap, where the scientists could measure transition frequencies of the electrons in the thorium ion with precision. Since electron energies are directly influenced by the nucleus of the atom, this gave Peik and his colleagues insight into how the nucleus of the thorium-229 atom will behave during a transition provoked by an ultraviolet laser.So while the needle in the haystack—that is, the correct UV frequency to excite a thorium-229 nucleus—hasn’t yet been found, Peik and his colleagues now have a picture of what the needle looks like. That way, they’ll know it when they see it.Read More: The Physicist Building the World’s Most Precise Clock